In the context of particle physics detection, LiquidO is a class of particle detection technology utilising opaque media for its ultimate light detection. While this may sound counterintuitive, this leads to a phenomenon, called stochastic light confinement (pioneered by LiquidO) enabling it to detect and generate images of subatomic fundamental particles, charged and neutral, as they deposit their energies in the medium while providing detailed information on event topology in the order of a few centimetre scales and vertex precision of up to a few millimetres. The imaging ability is designed to enable the identification of the type of particles involved (i.e. particle-ID) with energies from as low as a fraction of the MeV; i.e. a typical energy scale for low-energy nuclear physics processes. An example of e-γ discrimination is illustrated below from our Nature’s Communication Physics 4 273 2021. LiquidO detection capabilities are expected to improve at higher energies, such as GeV and above, as the integral light yield density increases drastically. It is well known that moving charged particle deposits energy in the trespassing medium causing emission of light due to Cherenkov and/or scintillation radiation mechanisms. Both are exploited by LiquidO for active detection.
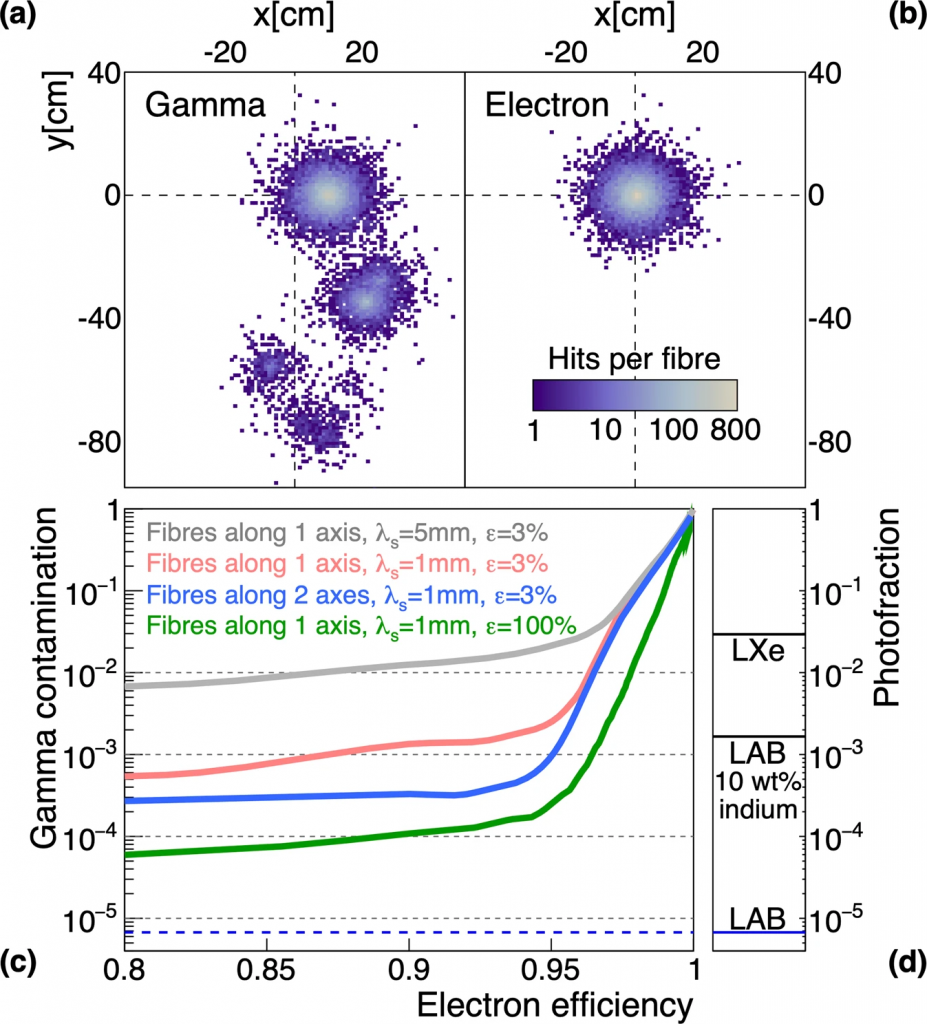
Historically, LiquidO was conceived in the context of neutrino fundamental research aiming to yield possible advances in some very challenging neutrino detection scenarios. This potential is still under demonstration. Despite commonalities, when it comes to photon detection strategy, LiquidO takes the completely opposite route as compared to the traditional transparent detectors. Indeed, most neutrino detectors so far have largely relied on the detection principle pioneered by Cowan & Reines et al. in the ’50s, upon the discovery of the neutrino, where the technology features the impeccable transparency of the detection medium so that light may propagate freely for optimal light detection. This approach has dominated neutrino detection for about 70 years with extreme success, including several Nobel prizes, including that for the discovery of the neutrino oscillations phenomenon (2015). LiquidO, in stark contrast, requires a strong level of opacity. So, its defining characteristic is the use of a detection medium enabling both the highest possible light emission and very high light scattering, thus drastically affecting the propagation of photons within the detector. This is further explained in the detection principle.
The LiquidO technique however may be applied to a vast range of fields where the detection of fundamental particle radiation is deemed necessary, such as Particle Physics, Astroparticle Physics, Nuclear Physics, High Energy Astrophysics, and Nuclear Medical Physics. Some of that is already actively being explored conceptually but also experimentally by the LiquidO consortium, along with some other scientists. Our focus is on both fundamental particle physics research and related innovation. The latter implies that emphasis is given to the application of technology to industrial or societal needs for a potential imminent technological transfer. Several projects are pursued, along with the corresponding R&D to ensure the readiness and optimisation of the technology, which is today considered to have reached a TRL of, at least, 5, upon the latest reported prototype results.
Most LiquidO detectors so far rely on scintillation for light production, as our interest remains in detection from the MeV scale upwards. If LiquidO worked successfully in the MeV scale, its performance is expected to largely suffice for the GeV scale, where some adjusting may be possible to enhance cost-effective constraints. Hence, a significant effort is geared to push the field of opaque scintillation, a technology “niche” being pioneered in the context of LiquidO since opacity is generally considered a very undesirable feature – to be avoided – in neutrino detectors in general. The implications may be so severe that, for example, famous experiments, like the ’90s CHOOZ, ended data-taking due to the uncontrolled loss of transparency in its liquid scintillator medium caused by chemical instability. LiquidO is being designed not only to mitigate these kinds of issues but even to possibly take bold advantage of the natural and abundant opacity to explore new novel detection media.
Naming Convention
The name “LiquidO” is decomposed into “Liquid” and “O“, with the “O” to be emphasised upon pronunciation. The former term (“Liquid”) refers to the historical context of liquid media used – and always favoured – for the construction of neutrino detectors, typically radiopure, large and to be built underground, while light emission can be readily produced via Cherenkov and scintillation radiation(s). The latter term (“O”) refers to the core opacity ingredient exploited here for detection for the first time.
While the technology naming may (misleadingly) suggest working “only in a liquid medium”, LiquidO may in principle work in any type of medium state (solid, liquid or gas), providing that the opacity constraint is practically met. In fact, LiquidO R&D has already been successfully demonstrated in the context of both solid and liquid phases, as reported by the results of our prototypes. Indeed, it turns out that the naming LiquidO does not fully represent the unanticipated apparent vast versatility of the technology – c’est la vie!
The name LiquidO is used for the technology and the consortium in the context of fundamental research. However, the projects using LiquidO technology in the context of innovation are further tagged OTech.